Ali Alnasif is a PhD student at Cardiff University. His project aims to develop a kinetic reaction mechanism for ammonia combustion characterised by its broad predictive accuracy across various conditions.
In this blog he explains the aims and methodology for his study: “Improving kinetic reaction mechanism for the complex conditions of engine chambers and the effects of turbulent flows.”
The combustion of fuels plays a crucial role in energy generation, powering everything from domestic tasks to industrial operations. Currently, 80% of global energy comes from the combustion of fossil fuels, which while effective, produces harmful pollutants. Carbon dioxide (CO2), the primary greenhouse gas, has been responsible for 78% of the global warming effect since 1990. Therefore, replacing conventional fuels to achieve zero carbon emissions is essential.
In response, clean technologies such as wind, solar, marine energy and biomass-based hydrogen have been proposed as net-zero alternatives. These solutions aim to reduce emissions and mitigate the energy sector’s impact on climate change, playing a key role in the transition to a sustainable, low-carbon future.
Energy storage options
Integrating renewable technologies into the grid faces challenges due to cost and transmission inefficiencies. Energy storage offers a practical solution, enabling the capture and storage of energy for later use or at different locations. Several storage techniques are available, with the choice depending on location, time of use and technology maturity.
Meanwhile, ammonia (NH3) has emerged as a promising energy carrier and chemical storage solution. It can support power generation at gigawatt scales and can be stored for extended periods without significant losses, provided storage conditions remain stable.
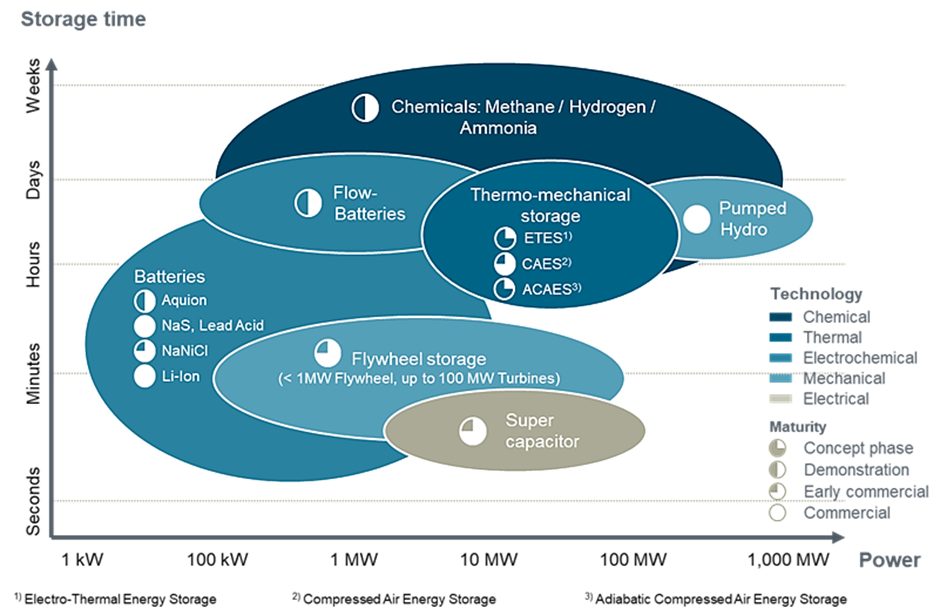
Figure 1: Energy storage technologies comparison. Reproduced from (Wilkinson, 2017).
Ammonia – pros and cons
Ammonia offers several advantages as a carbon-free fuel. It produces no direct CO₂ emissions which makes it ideal for decarbonising industries like shipping, and its established infrastructure for production, storage, and transportation makes it easier to scale compared to other alternatives.
However, harnessing ammonia as a fuel presents challenges due to its low flammability; but blending it with hydrogen improves combustion. Burning ammonia produces nitrogen oxides (NOx), which are harmful to health and the environment by contributing to air pollution and the greenhouse effect, so worsening climate change.
Fine-tuning ammonia combustion
Understanding ammonia’s combustion characteristics, tied to its molecular structure and kinetic behaviour, is crucial. To address these challenges, it is essential to study the fuel structure and the fundamental kinetics to develop a detailed chemical kinetic reaction mechanism that specifies the species involved and the reactions occurring during combustion on the molecular level.
The developed mechanism identifies key intermediates, reactants and products, mapping out pathways that lead to the formation of emissions such as NOx. By understanding how different species interact and the rates at which reactions occur, the combustion characteristics of ammonia can be controlled in real world applications to minimise pollutants.
Developing a kinetic reaction mechanism
My study aims to develop a kinetic reaction mechanism for NH3/O2 and NH3/H2/O2 flames under various conditions, ensuring efficient computational fluid dynamics (CFD) simulations under complex engine chamber conditions and turbulent flow dynamics.
By doing so, it will help identify the root causes of NOx formation and will lead to the development of novel strategies for reducing emissions. Ultimately, this detailed approach can unlock the potential of ammonia as an energy vector and provide solutions that make ammonia a more environmentally friendly and viable fuel for power generation applications, as shown below.
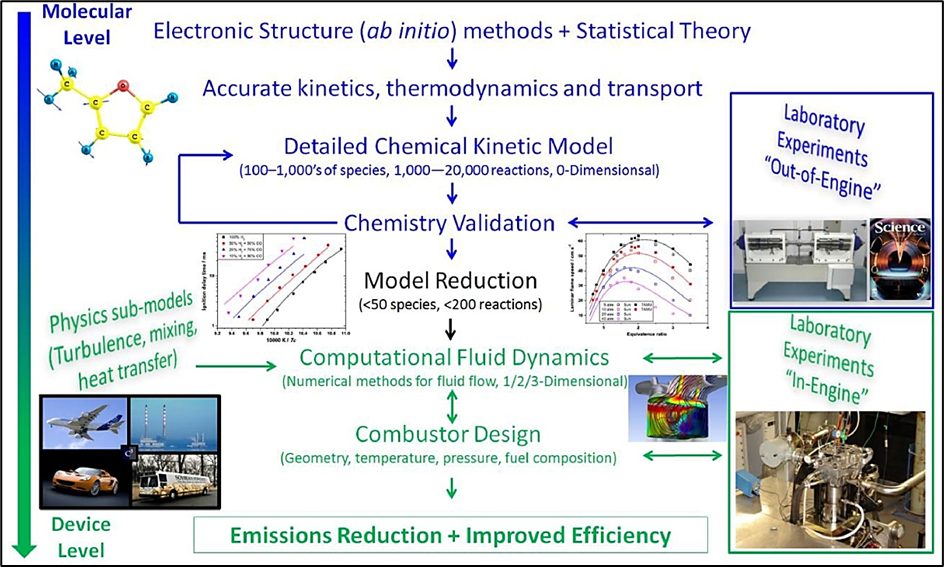
Figure 2: How combustion reactions at the molecular level affect the operation of modern combustors in practical applications. Reproduced from (Curran, 2019).
How the study will be undertaken
A chemical kinetic mechanism includes species with their thermodynamic and transport properties, as well as elementary chemical reactions with corresponding rate constants that describe the combustion process in detail.
The outcomes of the kinetic reaction mechanism are used to express the combustion characteristics of ammonia, including flame speed, ignition delay time, and NOx concentration. These results are obtained by running the mechanism in a flame simulator software application, such as Chemkin, to test its performance in a numerical model that mimics the combustion process in practical devices like jet-stirred reactors and burner-stabilised flames.
To improve accuracy, the mechanism is refined over time using experimental measurements from different combustion systems, which incorporate their uncertainty.
Optima++ is employed as a tool to adjust the kinetic model performance based on experimental data fed into the optimisation process. Improving the reaction mechanism indicates its readiness for validating experimental data across various combustion systems, helping to explore the underlying chemistry and find solutions to challenges like NOx emissions or enhancing flame speed.
Finally, applying this refined mechanism to CFD simulations for devices such as swirl burners or reciprocating engines can improve fuel efficiency and optimise combustor design.
So, the methodology can be summarised as:
- Collect experimental measurements from various combustion systems and conditions found in current literature to use as input targets for the optimisation process.
- Select an initial kinetic reaction mechanism that covers most of the required chemistry for the combustion process of ammonia.
- Identify the most influential reactions under different conditions.
- Obtain rate coefficients and their parameters (A, n, Ea) for the most influential reactions from the literature to be used in the optimisation process.
- Use the Optima++ code to determine the optimal set of parameters that best align with the input targets (experimental measurements).
- Apply the improved mechanism to CFD simulations to test its performance in a lab-scale generic swirl burner in the thermo-fluid labs at Cardiff University.
The resulting data should provide insights into the performance of the combustion process, focusing on combustion efficiency and key factors for improvement. This will help make the burner suitable for small, medium, or potentially large-scale power production.
A simplified sketch for the process is shown below:
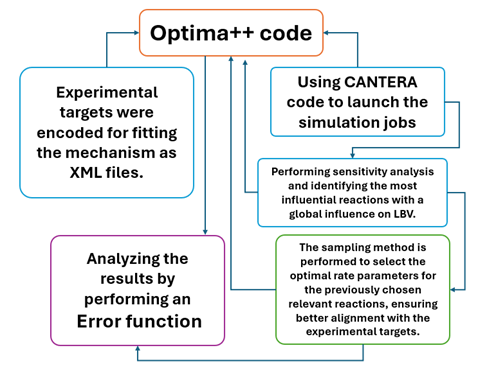
Figure 3: A simplified flowchart of the optimisation process
References
- Curran, H.J., 2019. Developing detailed chemical kinetic mechanisms for fuel combustion. Proceedings of the Combustion Institute 37, 57–81. https://doi.org/10.1016/j.proci.2018.06.054
- Wilkinson, I., 2017. Siemens green Ammonia. 1st NH3 European event. Netherlands: Rotterdam.